API Specification 5DP Drill Pipe: A Comprehensive Guide
Introduction API Specification 5DP Drill Pipe
Drill pipes are crucial components in the oil and gas industry, forming the backbone of drilling operations. These pipes connect the drilling rig to the drill bit, transmitting power and drilling fluid to create boreholes on the earth’s surface. This blog provides a detailed exploration of API Specification 5DP Drill Pipes, including their manufacturing process, types, connections, grades, and more. The goal is to equip you with practical knowledge and solutions to help you navigate the complexities of using drill pipes effectively.
What is API Specification 5DP Drill Pipe?
A drill pipe is a heavy, seamless, hollow tube that rotates the drill bit and circulates drilling fluid during drilling operations. It is designed to withstand significant stresses, including torsion, tension, and pressure while being lightweight enough to handle a rig easily.
Critical Functions of Drill Pipes:
- Transmission of Power: Drill pipes transfer the rotary motion from the drilling rig to the drill bit.
- Circulation of Drilling Fluid: They allow the circulation of drilling mud, which cools the bit, carries cuttings to the surface, and stabilizes the borehole.
- Lengthening the Drill String: As drilling progresses, additional drill pipe sections are added to the drill string to reach greater depths.
Manufacturing Process of API Specification 5DP Drill Pipe
The manufacturing of drill pipes is a highly controlled process designed to ensure the final product meets the stringent standards required for drilling operations.
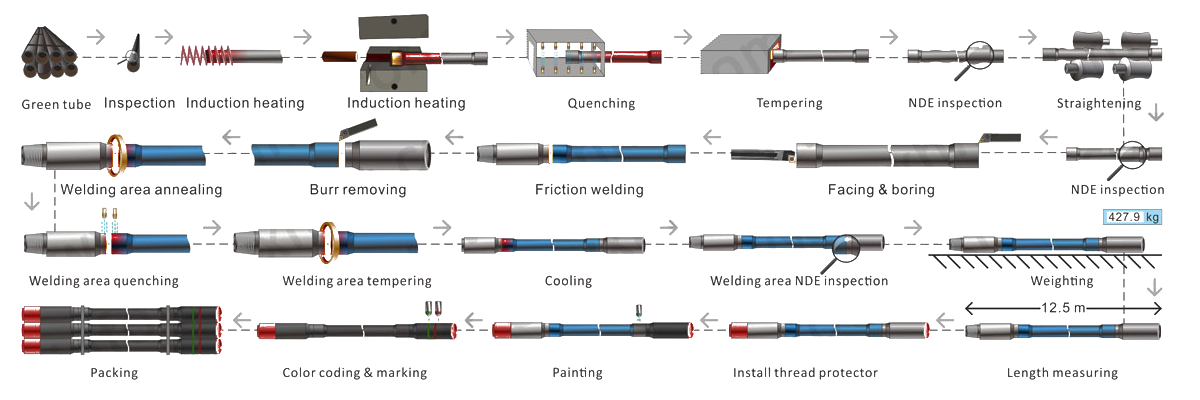
Manufacturing Process of Drill Pipe
1. Material Selection
- High-Quality Steel: The process begins with selecting high-grade steel, typically alloy steel such as AISI 4130 or 4140, known for its high strength and toughness.
- Chemical Composition: The steel’s composition is carefully controlled to achieve the desired mechanical properties, including resistance to wear, fatigue, and corrosion.
2. Pipe Forming
- Seamless Manufacturing: The steel is heated and pierced to create a hollow tube, which is elongated and rolled to form the drill pipe body.
- Welding (Optional): For certain types, steel plates may be rolled and welded to create the pipe.
3. Heat Treatment
- Quenching and Tempering: The pipes undergo heat treatment to enhance their mechanical properties, ensuring they can withstand the rigors of drilling.
4. Upsetting
- End Upsetting: The ends of the pipe are thickened to increase their strength. This process, known as upsetting, is crucial for enhancing the pipe’s durability at the connections.
5. Tool Joint Welding
- Attachment of Tool Joints: Tool joints are welded to the ends of the pipe, forming the connections that link each section of the drill string.
6. Hardbanding
- Wear-Resistant Coating: A wear-resistant alloy is applied to the tool joints to protect them from wear and extend the pipe’s service life.
7. Inspection and Testing
- Non-Destructive Testing: Each drill pipe undergoes rigorous testing, including ultrasonic and magnetic particle inspection, to ensure no defects.
- Dimensional Inspection: The pipes are measured to meet the required specifications.
8. Marking and Coating
- Identification: Each pipe is marked with essential information, such as grade, size, and manufacturer.
- Protective Coating: A corrosion-resistant coating is applied to the pipes to protect them during transportation and storage.
Types of API Specification 5DP Drill Pipe
There are several types of drill pipes, each designed for specific applications:
1. Standard Drill Pipe
- Description: The most common type of drill pipe used for standard drilling operations.
- Application: Suitable for conventional drilling in onshore and offshore environments.
2. Heavy Weight Drill Pipe (HWDP)
- Description: Thicker and heavier than standard drill pipe, HWDP is designed to add weight to the drill string, reducing buckling and improving stability.
- Application: Ideal for directional drilling and extended-reach wells.
3. Spiral Drill Pipe
- Description: This type features a spiral groove that reduces friction and wear during drilling.
- Application: Used in operations where friction reduction is critical.
4. Square Drill Pipe
- Description: A less common type with a square cross-section, offering increased rigidity.
- Application: Used in specific drilling scenarios requiring a rigid drill string.
5. Hexagonal Drill Pipe
- Description: Similar to the square drill pipe but with a hexagonal cross-section, providing enhanced torsional strength.
- Application: Suitable for high-torque drilling operations.
What are the Ends Processes of API Specification 5DP Drill Pipe?
In the context of drill pipes, the terms IU, EU, and IEU refer to different end processes that prepare the ends of the drill pipes for connections. These processes are crucial for ensuring that the drill pipe ends are durable, properly aligned, and suitable for threading and connection to other components in the drill string.
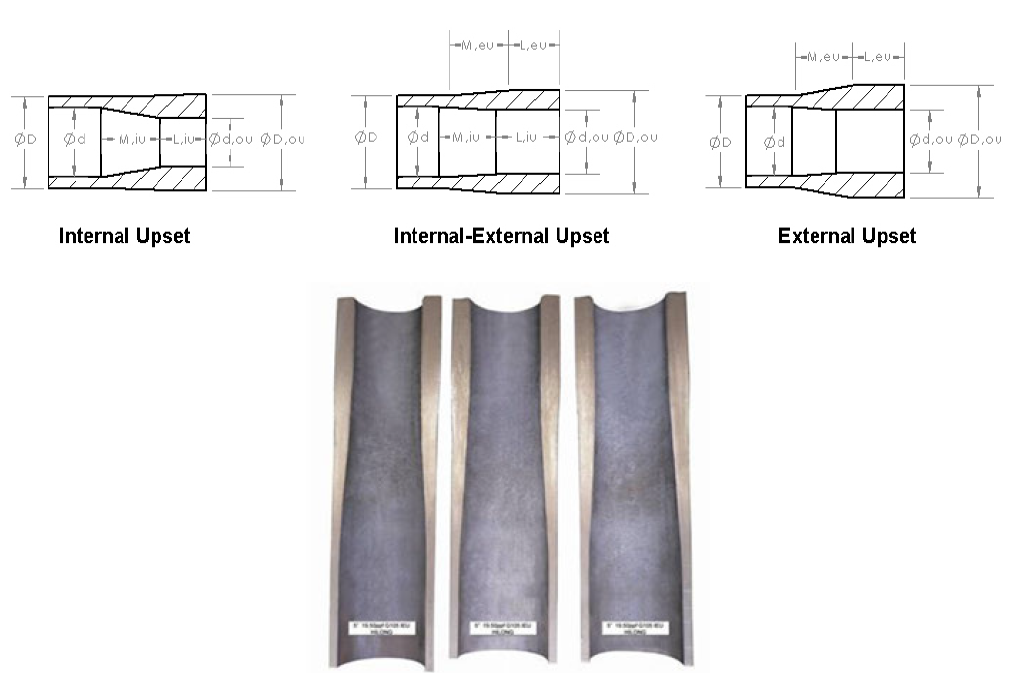
IU EU IEU of Drill Pipe Ends
1. Internal Upset (IU)
- Description: In an Internal Upset (IU) process, the pipe’s internal diameter is reduced, creating a thicker wall at the ends of the pipe.
- Purpose: This thickening increases the strength of the pipe ends, making them more resistant to the stresses and wear encountered during drilling operations.
- Application: IU pipes are used in situations where the internal diameter of the drill pipe is critical, such as in high-pressure drilling operations where maintaining a consistent bore is essential.
2. External Upset (EU)
- Description: External Upset (EU) involves increasing the pipe wall’s thickness at the pipe ends’ external diameter.
- Purpose: This process strengthens the pipe ends and enhances their durability, especially in areas where the drill pipe is most likely to experience wear and impact.
- Application: EU drill pipes are commonly used in standard drilling operations where external strength and impact resistance are prioritized.
3. Internal-External Upset (IEU)
- Description: Internal-External Upset (IEU) combines internal and external upsets, where the pipe ends are thickened internally and externally.
- Purpose: This dual-thickening process provides maximum strength and durability at the end of the drill pipe, offering enhanced resistance to internal and external forces.
- Application: IEU pipes are typically used in more demanding drilling environments, such as deep wells, high-pressure scenarios, and directional drilling, where internal and external reinforcement is needed.
Connections of API Specification 5DP Drill Pipe Tool Joints
The connections between drill pipe sections are critical for maintaining the integrity of the drill string. API 5DP drill pipes feature various types of connections:
1. Internal Flush (IF) Connection
- Description: Designed with a flush internal profile to minimize pressure drops and turbulence.
- Application: Used in high-pressure drilling environments.
2. Full Hole (FH) Connection
- Description: Features a larger bore for improved fluid flow, making it suitable for deep wells.
- Application: Ideal for deep drilling operations.
3. API Regular (API REG) Connection
- Description: A standard connection type known for its robustness and ease of use.
- Application: Commonly used in standard drilling operations.
4. Numerical Connection (NC)
- Description: A premium connection with high torque capacity, often featuring a double-shoulder design.
- Application: Suitable for challenging drilling conditions.
What are the Pin and Box in API Specification 5DP Drill Pipe?
Pin and Box refer to the two complementary ends of a drill pipe connection that allow the pipe sections to be securely joined together in a drilling string. This connection system is critical for maintaining the integrity and stability of the drill string during drilling operations.
Pin
- Description: The Pin is the male end of the connection. It is tapered and threaded, allowing it to be screwed into the Box.
- Design: The external threads of the Pin are precision-cut to match the internal threads of the Box, ensuring a tight, secure fit.
- Function: The Pin is designed to connect securely with the Box, creating a robust and leak-proof joint that can withstand the high pressures, torsional forces, and vibrations experienced during drilling.
Box
- Description: The Box is the female end of the connection. It is also threaded internally to accommodate the Pin.
- Design: The Box’s internal threads are precisely machined to match the Pin’s threads, allowing for a secure and tight connection.
- Function: The Box receives the Pin, creating a sturdy connection that ensures the drill pipe sections remain connected and aligned during drilling operations.
Importance of Pin and Box Connections
- Structural Integrity: The Pin and Box connection ensures the drill pipe sections are securely fastened, maintaining the structural integrity of the drill string.
- Pressure Resistance: These connections are designed to withstand the high internal pressures generated by drilling fluid circulation.
- Ease of Use: Pin and Box connections are designed for easy assembly and disassembly, facilitating quick changes and adjustments to the drill string.
Applications
- Drill Pipes: Pin and Box connections are used in all drill pipes, including standard, heavy-weight, and specialized pipes.
- Tool Joints: These connections are also used in tool joints, which are thicker, heavier sections of drill pipes that provide added strength and durability.
Grades, Diameters, Length Ranges, and Applications
Drill pipes come in various grades, diameters, and lengths, each suited to different drilling environments:
Grades
- E-75: Commonly used for general drilling operations.
- X-95: Provides higher strength and is suitable for deeper wells.
- G-105: Offers excellent fatigue resistance, ideal for extended-reach drilling.
- S-135: The highest strength grade, used in ultra-deep and high-pressure wells.
Diameters and Lengths
- Diameters: Typically range from 2 3/8″ to 6 5/8″.
- Lengths: Range from 27 to 31 feet, with custom lengths available based on project needs.
Applications by Grade
- E-75: Onshore drilling in standard conditions.
- X-95: Deep wells with moderate pressures.
- G-105: Extended-reach wells and high-torque drilling.
- S-135: Ultra-deep, high-pressure, and high-temperature wells.
Packing, Storage, Maintenance, and Transportation
Proper handling of drill pipes is crucial for maintaining their integrity and extending their service life.
Packing
- Bundling: Drill pipes are typically bundled together for easier handling and transportation.
- Protective Caps: Both ends of the drill pipe are fitted with protective caps to prevent damage to the threads.
Storage
- Indoor Storage: Whenever possible, drill pipes should be stored indoors to protect them from the elements.
- Elevated Storage: Pipes should be stored off the ground on racks to prevent contact with moisture and contaminants.
Maintenance
- Regular Inspections: Drill pipes should be inspected regularly for signs of wear, corrosion, or damage.
- Re-threading: Threads should be re-cut if damaged, ensuring a secure connection.
Transportation
- Secure Loading: Drill pipes should be securely loaded onto trucks or trailers to prevent movement during transit.
- Use of Cradles: Pipes should be transported using cradles to prevent bending or damage.
Conclusion
API Specification 5DP Drill Pipe is a critical component in drilling operations, designed to withstand the harsh conditions encountered during oil and gas extraction. Understanding the manufacturing process, types, connections, grades, and handling of drill pipes is essential for optimizing their performance and ensuring safe, efficient drilling operations.
By following best practices in selecting, storing, and maintaining drill pipes, operators can extend the life of their equipment, reduce operational costs, and minimize the risk of failures. This comprehensive guide is a valuable resource for professionals in the drilling industry, offering practical insights and solutions to the challenges associated with drill pipes.