Submarine Pipeline: An In-Depth Exploration
Introduction
Submarine pipelines transport oil, gas, and other fluids across vast underwater distances. These pipelines are essential for connecting offshore oil and gas platforms to onshore facilities, enabling the efficient and safe transfer of resources from remote locations to the global market. However, designing, constructing, and maintaining submarine pipelines present unique challenges due to the harsh and dynamic marine environment. This blog provides a comprehensive overview of submarine pipelines, including their applications, design considerations, materials, installation methods, and maintenance strategies.
1. The Role of Submarine Pipeline in the Energy Industry
1.1 Importance of Submarine Pipelines
Submarine pipelines are the lifelines of the offshore oil and gas industry. They provide a direct and secure route for transporting hydrocarbons from offshore fields to refineries and processing plants onshore. These pipelines are essential for the global energy supply chain, particularly as the demand for oil and gas grows.
1.2 Key Applications
Oil and Gas Transportation: Submarine pipelines are primarily used to transport crude oil, natural gas, and condensates from offshore production platforms to onshore facilities for processing and distribution.
Water Injection Lines: In enhanced oil recovery (EOR) operations, submarine pipelines inject water into the reservoir to maintain pressure and improve oil recovery rates.
Subsea Umbilicals: These specialized pipelines carry control fluids, electrical power, and communication signals between subsea equipment and topside facilities.
2. Design Considerations for Submarine Pipeline
2.1 Environmental and Geotechnical Factors
Marine Environment: Submarine pipelines must be designed to withstand the corrosive effects of seawater, strong currents, and potential impacts from marine vessels or objects. The temperature and pressure conditions at the seabed also influence material selection and pipeline design.
Seabed Topography: The topography of the seabed, including slopes, valleys, and trenches, affects pipeline routing and stability. Geotechnical surveys are essential to assess the seabed conditions and determine the best pipeline route.
Seismic Activity: In regions prone to earthquakes, submarine pipelines must be designed to accommodate ground movement and prevent damage during seismic events.
2.2 Pipeline Integrity and Safety
Material Selection: Submarine pipelines are typically constructed from high-strength carbon steel, coated with anti-corrosion materials such as fusion-bonded epoxy (FBE) or three-layer polyethylene (3LPE) to protect against corrosion and mechanical damage.
Pipeline Stability: Stability is a critical concern, particularly in areas with strong currents or uneven seabed terrain. Techniques like trenching, burying, or adding concrete weight coatings stabilize the pipeline.
Leak Detection Systems: Submarine pipelines are equipped with advanced leak detection systems that monitor pressure, flow rates, and other parameters in real-time to detect leaks or anomalies.
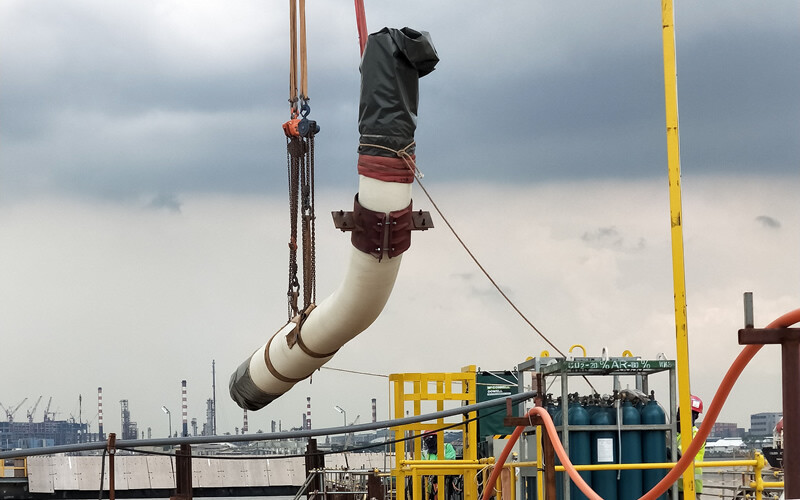
Subsea or Submarine Pipeline
3. Materials Used in Submarine Pipeline Construction
3.1 Carbon Steel
Carbon steel is the most common material for submarine pipelines due to its strength, durability, and cost-effectiveness. It is typically coated with anti-corrosion layers to protect against the harsh marine environment.
3.2 Corrosion Resistant Alloys (CRAs)
In areas where pipelines are exposed to highly corrosive fluids, such as sour gas or seawater, corrosion-resistant alloys like stainless steel, duplex stainless steel, or Inconel are used. These materials offer excellent resistance to corrosion and high mechanical strength.
3.3 Coating Systems
Fusion-Bonded Epoxy (FBE): FBE coatings provide excellent corrosion protection and are commonly used for submarine pipelines.
Three-Layer Polyethylene (3LPE): 3LPE coatings consist of an epoxy primer, a polymer adhesive, and a polyethylene topcoat, offering superior mechanical protection and corrosion resistance.
Concrete Weight Coating: Concrete weight coatings are often applied to ensure stability and protect the pipeline from external damage, especially in areas with strong currents or where the pipeline is laid on the seabed.
4. Installation Methods
4.1 S-Lay Method
The S-lay method is one of the most common techniques for installing submarine pipelines. The pipeline is welded on a lay barge and gradually lowered to the seabed in an S-shaped curve. This method is suitable for shallow to medium water depths.
4.2 J-Lay Method
In the J-lay method, the pipeline is lowered vertically into the water and laid horizontally on the seabed. This technique is ideal for deepwater installations, as it reduces stress on the pipeline during the laying process.
4.3 Reel-Lay Method
The reel-lay method involves spooling the pipeline onto a large reel and then unspooling it during installation. This fast and efficient method makes it suitable for short pipelines or installations in remote locations.
4.4 Trenching and Burial
In areas with high environmental risk or where seabed stability is a concern, the pipeline is buried in a trench to provide additional protection against external forces. Trenching can be performed using plows, jetting tools, or mechanical trenchers.
5. Maintenance and Integrity Management
5.1 Inspection Techniques
In-Line Inspection (ILI): ILI tools, commonly known as “smart pigs,” are used to inspect the internal condition of submarine pipelines. These tools can detect corrosion, cracks, and other anomalies that may compromise pipeline integrity.
ROV and AUV Inspections: Remotely operated vehicles (ROVs) and autonomous underwater vehicles (AUVs) inspect the external condition of submarine pipelines. These vehicles have cameras and sensors to monitor the pipeline’s condition and identify potential issues.
5.2 Corrosion Monitoring
Cathodic Protection: Cathodic protection systems prevent corrosion on the external surface of submarine pipelines. These systems use sacrificial anodes or impressed currents to protect the pipeline from corrosive seawater.
Corrosion Inhibitors: In some cases, corrosion inhibitors are injected into the pipeline to protect the internal surfaces from corrosive fluids.
5.3 Leak Detection and Emergency Response
Real-Time Monitoring: Submarine pipelines have real-time monitoring systems that detect leaks, pressure drops, and other anomalies. Early detection is critical to preventing environmental damage and ensuring the pipeline’s safety.
Emergency Response Plans: Comprehensive emergency response plans are in place to address potential leaks or ruptures in submarine pipelines. These plans include procedures for shutting down the pipeline, containing the leak, and mitigating environmental impact.
6. Conclusion: The Future of Submarine Pipeline
As energy demand continues to grow, submarine pipelines’ importance in the global energy supply chain cannot be overstated. Advances in materials, installation techniques, and integrity management are enhancing the reliability and safety of these critical infrastructures. However, ongoing challenges such as corrosion, environmental impact, and deeper water installations require continuous innovation and improvement.
By understanding the complexities of submarine pipelines and adopting best practices in design, construction, and maintenance, the industry can ensure the safe and efficient transport of resources from offshore fields to markets worldwide.
7. FAQs
Q1: What are the main challenges in submarine pipeline construction?
The main challenges include environmental factors such as corrosion, strong currents, seabed stability, and technical challenges related to material selection, installation methods, and maintaining pipeline integrity over time.
Q2: How are submarine pipelines protected from corrosion?
Submarine pipelines are protected from corrosion through the use of anti-corrosion coatings such as FBE and 3LPE, cathodic protection systems, and corrosion-resistant materials in highly corrosive environments.
Q3: What is the importance of real-time monitoring in submarine pipelines?
Real-time monitoring is crucial for detecting leaks, pressure drops, and other anomalies that could compromise pipeline integrity. Early detection allows for quick response and mitigation, preventing environmental damage and ensuring pipeline safety.